Integrated Modeling 101: Training Module
PDF version of this training | All modeling training modules
Integrated Modeling 101
Building upon the concepts presented in the other Council for Regulatory Environmental Modeling (CREM) training modules, this module focuses on integrated modeling.
The module introduces the concepts of integrated modeling, provides illustrative case studies and examples of integrated modeling efforts and outlines the role of this approach in facilitating integrative systems analysis and decision making.
This module is designed to be stand-alone; a basic understanding of the concepts and terminology covered in other modules is assumed. For review, please consult the CREM Guidance Document (EPA, 2009b) or these other modules:
Additional Web Resources:
Fundamental topics of environmental modeling are covered in other modules:
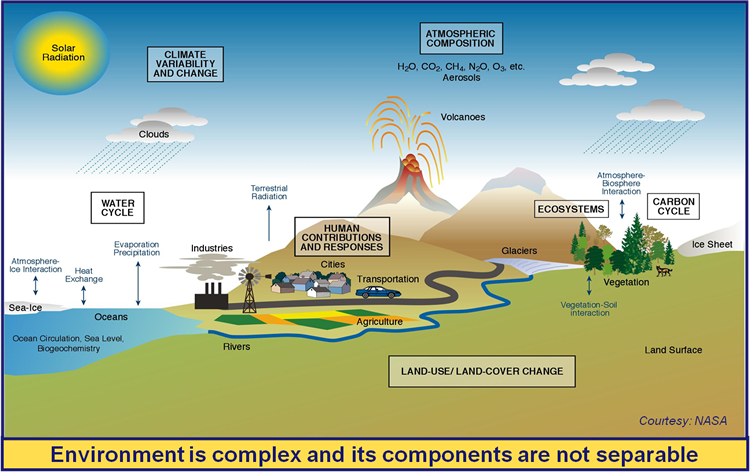
Modeling Complex and Interrelated Systems: Our surrounding environment is made up of many systems, processes and cycles that cross many media; all affected by our interaction with the environment. Integrated modeling is one approach for evaluating how these systems interact. Figure adapted from NASA. (Click on image for a larger version)
What is Integrated Modeling?
EPA (2008; 2009b) defines integrated modeling as:
"A systems analysis-based approach to environmental assessment. It includes a set of interdependent science based components (models, data, and assessment methods) that together form the basis for constructing an appropriate modeling system. The constructed modeling system is capable of simulating the environmental stressor-response relationships relevant to a well specified problem statement."
Integrated modeling can be considered linking an individual modelmodelA mathematical representation of a natural system intended to mimic the behavior of the real system, allowing description of empirical data and predictions about untested states of the system. together linearly or by identifying co-dependencies among models (please see the Integrated Thinking subtab at the end of this section). However, integrated modeling systems go beyond the linking of two or more models; it also involves the IT infrastructure required to handle the data, information architectures, evaluation of the results, etc. (see the Computational Platforms and Summary sections).
We will make a distinction in this module between computation platforms for integrated models and integrated models.
Important Environmental Issues: Aspects of model integration that should be considered when tackling environmental problems. Adapted from Parker et al. (2002).
Why is Integrated Modeling Necessary?
Our environment is complex and the science necessary to understand its processes encompasses many disciplines (e.g. biological sciences, chemistry, physical sciences, ecology, economics, human behavior, etc.) that are typically isolated from one another. Similarly, the environmental policies coming out of the decision making process are often cross-disciplinary, requiring a more holistic approach of the modeling done to inform our decisions.
Therefore, the reasons for integrated modeling and a systems thinking approach can be grouped into:
- Science Based Drivers
- Policy Based Drivers
Finally, integrated modeling steps away from typical stove piped approaches to support integrated decision making that supports sustainablility.
Science Based Drivers for Integrated Modeling:
Trans-disciplinary integrated modeling extends beyond the environmental sciences to include other disciplines such as economics, human behavior, land-use, valuation of ecosystem services, etc.
For example, a conventional stove-piped approach may result in impacts in other sectors that were not considered in the scope of the problem. An integrated transdisciplinary or systems thinking approach typically broadens the scope of the problem, thus including other sectors where unintended impacts (negative or positive) would be identified.
Policy Based Drivers for Integrated Modeling:
Future policy-making may place greater demands on the models often used to inform them. Decisions often consider future scenarios for alternative policy implications. Therefore integrated models should have the ability to forecast future scenarios (e.g. climate) based upon alternative policy decisions. In general, modeling has been shown to be a useful tool for informing the decision making process. Integrated modeling has the ability to take current modeling capabilities and apply them in a context that is more informative, more systems based, and coordinated across many sectors.
Policy should be: | Modeling capability: |
---|---|
Forward-looking | Forecasting |
Coordinated | Integrated policy analysis |
Systems-based | Comprehensive/integrated |
Info-intensive | Produce useful output |
Incentive-based | Optimization analysis |
Integrated Decision Making:
The decision making process within each sector (e.g. economic, societal, environmental) is usually done independently, though the output from each sector is often considered during in overarching policies. There is often stove-piping within sectors (e.g. air, water and other media within the environmental sector). With integration, the sectors interact to form a strong foundation to the decision making process. A decision making process that integrates information from multiple sectors (models) could better inform policy decisions.
Benefits of Integrated Modeling (EPA, 2008):
The integrated modeling approach is applicable to a wide spectrum of environmental assessment and management needs. Investing in the development of an integrated modeling infrastructure may result in substantial returns, with efficiencies that benefit both the modeling and the decision making communities.
Examples of the types of benefits gained from integrated modeling approaches include those in the adjacent pane.
Tol and Vellinga (1998) also note that "the whole of integrated assessment should be greater than the sum of the disciplinary parts." In other words, integrating models should provide added information compared to an individual (or system specific) model.
Benefits of Integrated Modeling (EPA, 2008):
- Assess risk in multiple media by evaluating multiple sources, stressors, pathways and receptors;
- Address multidisciplinary questions, or those in which social, political, and economic factors might substantially influence decision making;
- Analyze systems where processes and systems are significantly linked through two-way (or greater) coupling and feedback;
- Clarify information for decision makers without resulting in an unacceptably high level of uncertainty;
- Link source information (emissions) to ambient concentrations (whether in air, water, or soil) and, finally, to human health or ecological effects;
- Conduct cost-benefit analysis and valuation of ecosystem services;
- Weigh many alternative regulatory or management scenarios;
- Develop adaptive management strategies;
- Identify potential sources of observed environmental degradation.
EPA's Strategic Vision for Integrated Environmental Decision Making
The US EPA published a white paper (EPA, 2008) that built upon a highly successful workshop organized by EPA’s CREM and the Office of Research and Development’s Ecosystems Research Division. This workshop aimed to initiate a broad-based dialogue on the use of integrated modeling approaches to inform environmental decision making.
The white paper proposes a strategic vision for integrated environmental decision making, supported with recommendations to achieve this strategic vision. Briefly, those recommendations included (EPA, 2008):
- Promotion and adoption of a systems approach to research efforts that support decision making
- Implementing modeling into the decision making process in a way that enhances the decision as well as stakeholder involvement
- Pursuing an organizational approach to promote integrated modeling, rather than project by project
Including integrated modeling in the decision making process could result in significant improvement in problem understanding and solution effectiveness as well as enhancing stakeholder collaboration and the transparency of decision making (EPA, 2008).
Additional Resources:
Integrated Modeling for Integrated Environmental Decision Making. 2008. EPA 100-R-08-010. Washington, DC. Office of the Science Advisor, US Environmental Protection Agency.
Integrated Modeling at EPA
Evolving regulatory focus of the US EPA from single pathway analyses to integrated modeling approaches (Whelan and Laniak, 1998; EPA, 2008).
Early Years at EPA "Single Pathway Analysis" |
Recent Past/Present "Multiple Pathway Analysis" |
Future "Integrated System Analysis |
---|---|---|
Single Chemical | Multiple Chemicals, sediments | Mixtures, sediments, land use change, climate change |
Multiple Chemical Sources | Multiple Chemical Sources | Multiple Chemical/Non-chemical Sources |
Single Medium Fate & Transport | Linked Media Fate & Transport | Integrated Multimedia Fate and Transport with Mass Balance and Feedback |
Single Exposure Pathway | Multiple Exposure Pathway Analysis | Integrated Exposure Pathway Analysis, Land Use Management |
Cancer Risk to Maximally Exposed Individual (MEI) | Cancer/Non-cancer Risk Across Human Population Ecological Risk (Populations) | Aggregate Cancer/Non-cancer Risk across Human Population Ecological Risk (populations, communities), Ecosystem health and services evaluation |
Deterministic Analysis![]() |
Probabilistic Analysis![]() |
Probabilistic Analysis, Adaptive Management Strategies |
Model Calibration![]() |
Model Calibration/Qualitative and Limited Quantitative Uncertainty | Comprehensive and Fully Integrated Uncertainty Analysis |
Aspects of Integrated Thinking
The various definitions related to integrated modeling underscore the important concepts of an integrated, or 'systems thinking' approach.
The terms associated with ‘integrated modeling’ are used inconsistently in the literature, often varying from discipline to discipline. In some instances, these definitions over-lap; further contributing to the confusion. A few of the more prominent terms are identified and summarized here:
- Integrated Modeling
- Integrated Assessment
- Integrated Assessment Modeling
- Integrated Environmental Modeling
- Integrated Environmental Decision Making
- Integrated Environmental Strategies
In any of these definitions, ‘integrated’ conveys a message of multi- or interdisciplinarity or ‘systems thinking’; and ‘assessment’ a message of policy relevance (Tol and Vellinga, 1998). Whereas ‘modeling’ indicates the development and/or application of simulation models.
Integrated Environmental Strategies (IES) (EPA, 2004)
The IES approach enables local researchers to quantify the co-benefits that could be derived from implementing policy, technology, and infrastructure measures to reduce air pollutants and GHG emissions. Thus,integrating environmental models with economic and health effect models to conduct a cost-benefits analysis of pollution control measures.
Integrated Assessment (Parson, 1995; Weyant et al., 1996; Jakeman and Letcher, 2003)
The two defining characteristics of integrated assessment are (a) that it seeks to provide information of use to some significant decision maker rather than merely advancing understanding for its own sake; and (b) that it brings together a broader set of areas, methods, styles of study, or degrees of certainty, than would typically characterize a study of the same issue within the bounds of a single research discipline. Briefly, integrated assessments link research to policy.
Integrated Assessment Modeling (Rotmans and van Asselt, 2001; Rosenberg and Edmonds, 2005)
Integrated assessment modeling is an analytical approach that brings together knowledge from a variety of disciplinary sources to describe the cause-effect relationships by studying the relevant interactions and cross-linkages. Recently, it has been applied to complex environmental issues; bringing together natural science, social science and economic dimensions.
Integrated Environmental Modeling
Integrated environmental modeling is a discipline of developing a system of models where models from two or more academic disciplines are integrated such that they behave like a unit to external stimuli. At least one of the models in the system is from environmental domain while others may come from other academic disciplines such as socio-economic domain. The models integrated into the system are usually developed in complete isolation from each other.
Integrated Environmental Decision Making (EPA, 2000)
Integrated decision making is a natural progression from fragmented approaches for protecting human health and the environment. Integrated thinking about complex environmental problems is at the core of this concept. The process also includes integrating resources and analyses to address the problems as they occur in the real world, and integrating input from the public and interested and affected parties.
Integrated Environmental Strategies (IES) (EPA, 2004)
The IES approach enables local researchers to quantify the co-benefits that could be derived from implementing policy, technology, and infrastructure measures to reduce air pollutants and GHG emissions. Thus,integrating environmental models with economic and health effect models to conduct a cost-benefits analysis of pollution control measures.
Conceptual Models of Integrated Systems
Similar to individual model development, an integrated modeling project should start with a conceptual modelconceptual modelA graphic depiction of the causal pathways linking sources and effects, that ultimately is used to communicate why some pathways are unlikely and others are very likely. of the system of interest.
A defining feature of integrated modeling is the holistic, or ‘systems thinking’ approach to solving a specific problem. Take for example the problem of hypoxia in the Northern Gulf of Mexico.
A conventional approach to characterizing the hypoxia problem might be to model pollutant loads coming from the rivers that feed the Gulf. Non-point and point sources may be identified and pollution controls could be used to alleviate loads delivered to the Gulf.
Alternatively, an integrated approach could also consider atmospheric sources, land-use changes, climate change impacts, and how different economic sectors and activities may influence the pollutant loads reaching the Gulf.
There are existing approaches which can help in the conceptualization of an integrated system. These conceptual models can help to identify how models addressing different processes can be integrated to an anaylsis of the whole system.
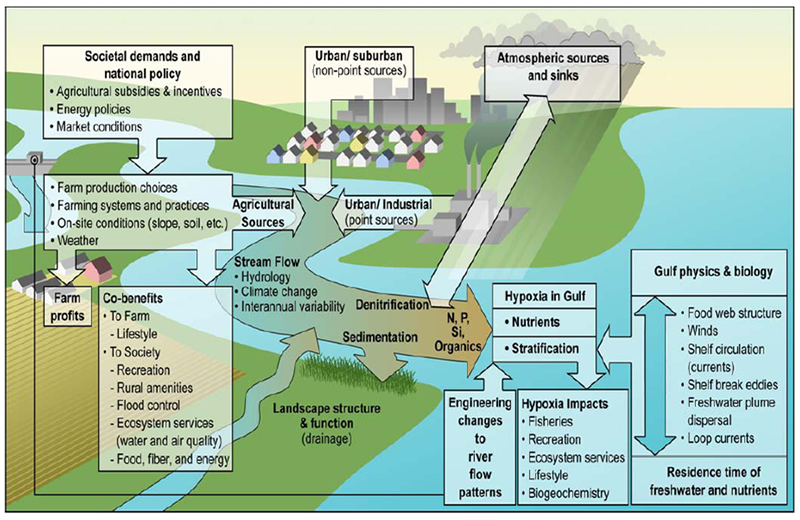
An Example of a Conceptual Model of an Integrated Approach: Complex environmental issues, like the hypoxia problem in the Northern Gulf of Mexico, may be better assessed with an integrated modeling approach utilizing information (models, data, etc.) from multiple sectors. Image adapted from EPA (2007). (Click on image for a larger version)
Driver-Pressure-State-Impact-Response
The DPSIR approach (Smeets and Weterings, 1999) is a conceptual tool that has been found useful for organizing and simplifying the complexity of ecosystem management (Ojeda-Martínez et al., 2009). As suggested by its name, DPSIR consists of five components that inform decision making:
- Drivers (D) represent societal needs such as food, water, shelter and cultural connection. These needs can be identified in economic sectors.
- Pressures (P) are created as a result of human activities (Drivers) on the environment. Natural resource use, land-use/land-cover change, transportation networks and release of chemicals are examples of pressures.
- The state (S) of the environment is subject to changes brought upon by the Pressures. Measures of environmental state include concentrations of pollutants in the air, water, soil and biota, human and ecosystem health status.
- Impacts (I) to the environment result from changes in the State; such as changes in ecosystem function and the delivery of environmental goods and services. Impacts can also manifest in human health and the socio-economic functioning of society.
- Responses (R) are actions taken by society, in response to an impact (e.g, regulations). These responses can address the drivers, pressures, states or impacts.
The DPSIR approach.
A DPSIR Example
The DPSIR approach can be applied to the development of a
TMDLTMDLCalculation of the maximum amount of a pollutant that a waterbody can receive and still meet water quality standards and an allocation of that amount to the pollutant's source. (Total Maximum Daily Load) conceptual model. For example, under the Clean Water Act, the development of a TMDL can be a response to an impact (non-attainment of designated use). This response is aimed at mediating a pressure (point- and non-point source pollutant or sediment loads) to effect a change in state (physical, chemical or biological characteristics of the water body).
The systems or sectors that are included in this approach include the agricultural (as non-point source of pollution), industry (point sources), environmental (plant and animal populations, ecosystem services), valuation of ecosystem services, and human behavior (recreation, waste disposal, etc.). Air circulation and weather models might also be included to account for deposition and precipitation.
Not all TMDLs are developed this way, this is just an example of how the DPSIR approach could be used to facilitate an integrated modeling approach.
Additional Web Resources
General information regarding the Agency's TMDL program can be found at:
Impaired Waters and Total Maximum Daily Loads
Driver-Pressure-State-Exposure-Effect-Action
Similar to DPSIR, the DPSEEA approach can help to organize information when developing an integrated modeling scheme. The approach describes six components of the environment-health chain including exposure (Smeets and Weterings, 1999; Briggs, 2003):
- Drivers (D) represent societal needs such as food, water, shelter and cultural connection. These needs can be identified in economic sectors.
- Pressures (P) are created as a result of human activities (Drivers) on the environment. Natural resource use, land-use/land-cover change, transportation networks and release of chemicals are examples of pressures.
- The state (S) of the environment is subject to changes brought upon by the Pressures. Measures of environmental state include concentrations of pollutants in the air, water, soil and biota, human and ecosystem health status.
- Exposures (E) — that take place when humans are exposed to these changed environmental conditions.
- Effects (E) — adverse impacts on health due to these exposures.
- Actions (A) — policy and other interventions, aimed at reducing or avoiding these adverse health effects.
A DPSEEA Example
The DPSEEA approach could be applied to address drinking water contamination. For example, drinking water sources, which all humans need, could become contaminated with toxic chemicals through a variety of drivers (e.g. land use changes, hydraulic fracking, and improper waste disposal). If contaminated water is ingested, generic health effects (e.g. morbidity or mortality) may develop. Appropriate actions can then be taken place along the chain to prevent further adverse health effects.
In this example, water quality models, risk assessment models, exposure models, and economic models may all be integrated to inform the policy actions.
Example Integrated Modeling Activities
In this section, a few integrated modeling activities are highlighted. This is not intended to be a comprehensive list, but rather provide some examples of integrated modeling systems from across the Agency.
Additional information for each of the examples is provided in next pages of the module.
Additional Web Resources
Chesapeake Bay Program:
NESCAUM:
BASINS:
Chesapeake Bay Program (CBP)
The essential water quality problem in the Chesapeake Bay is eutrophication; the over-enrichment of estuary waters that results in low bottom dissolved oxygen, excessive levels of algae, and poor water clarity.
The restoration effort is guided by three water quality standards: dissolved oxygen, chlorophyll, and water clarity. The Chesapeake Bay Program has been applying increasingly sophisticated integrated models to support restoration for more than two decades.
The first integrated models were relatively crude, being nothing more than a simple linkage of a watershed model and a model of the estuary. As the scope and sophistication of decision making grew in the Chesapeake, the integrated models being used now include models of the airshed, watershed, estuary, living resources, and climate change.
This information was adapted from the Chesapeake Bay Program website. Exit
The Chesapeake Bay Program Integrated Model: The CBP integrates models of the airshed, watershed, estuary water quality and sediment transport, key living resources, and climate change.
NESCAUM
Identifying Policy Options: Developing a Regional Integrated Energy-Environment Analytical Approach
There is great need for economic, energy and environmental information to support sound energy and air quality planning at the state and regional level. This information is required to assist decision-makers in understanding the challenges presented by multi-pollutant management options and regional policy commitments. The National Risk Management Research Lab in EPA’s Office of Research and Development has been supporting the development of an integrated analytical modeling framework for the Northeast States by the Northeast States for Coordinated Air Use Management (NESCAUM).
This analytical approach includes MARKet ALlocation technology database and model (NE-MARKAL), the REMSAD and CMAQ regional air quality models and the BenMAP and COBRA health benefits assessment tools. This analysis is being developed to support the New England Governors and Eastern Canadian Premiers (NEG/ECP) climate action planning, as well as other pressing regional issues (e.g. achieving and sustaining air quality standards).
Additional information on the models in this example.
NESCAUM Conceptual Model: The integrated regional policy analysis from the Northeast States by the Northeast States for Coordinated Air Use Management (NESCAUM). For updated information please see their Climate and Energy website. Exit Image adapted from EPA (2008).
The Integrated Models within NESCAUM
MARKAL
REMSAD
CMAQ
BenMAP
COBRA
BASINS - Better Assessment Science Integrating point and Nonpoint Sources
EPA's BASINS modeling integrates environmental data, analytical tools, and watershed modeling programs. BASINS supports the development of cost-effective approaches to watershed management and environmental protection (EPA, 2009a).
EPA developed the Climate Assessment Tool (CAT) for BASINS 4.0 in 2006. BASINS-CAT provides a flexible set of capabilities for modifying historical weather data to create climate change scenarios for assessing the influence of climate variability and change on water quantity and quality using the Hydrologic Simulation Program-FORTRAN (HSPF) watershed model.
The BASINS modeling system combines five components (EPA, 2009a):
- a comprehensive collection of national cartographic and environmental databases;
- environmental assessment tools (to summarize results; establish pollutant source/impact interrelationships; and selectively retrieve data);
- utilities (e.g. import tool, download tool, grid projector, post-processor, and land use, soil classification and overlay tool);
- automated watershed characterization reports (for eight different data types); and
- a suite of watershed models.
Multi-Use Computational Platforms
Within the integrated modeling community, there are development efforts to construct computational platforms which will allow for (relatively) seamless linking of individual, stand-alone models (they may be referred to as components).
These platforms serve as the backbone for large and complex integrated modeling efforts. They are designed to handle data storage/retrieval, data processing, synchronization of model runs, and other communications between the individual components/models, etc.
Some of the platforms have standards for maximized inter-operability (input/output protocols, operating platform, etc.); others allow the model developers a little more flexibility.
With computational platforms, independent models can be linked together much like puzzle pieces interconnect.
Open Modeling Interface and Environment
The Open Modeling Interface and Environment (OpenMI) defines standards for model linkage in the water domain. While not necessarily a platform, models that conform to this standard are thus compatible.
The main driver for the development of the OpenMI standard was the European Union’s Water Framework Directive that calls for an integrated approach to water management throughout Europe (Moore et al., 2007; EPA, 2008).
The OpenMI standard defines an interface that allows time-dependent models to exchange data at runtime. When the standard is implemented, existing models can be run in parallel and share information at each time-step. The features of OpenMI help to address the types of model integration described to the right.
Additional Web Resource:
The OpenMI Life project website Exit
Features of the OpenMI standard:
(Adapted from Moore et al., 2007; EPA, 2008)
- Link models from different domains (hydraulics, hydrology, ecology, etc.) and environments (atmospheric, freshwater, rural, etc.)
- Link models based on different modeling types (deterministic, stochastic, etc.)
- Link models of different dimensionality (0, 1, 2, 3D)
- Link models working at different scales (e.g. a regional climate model to a catchment runoff model)
- Link models operating at different temporal resolutions (e.g. hourly to monthly or even annual)
- Link models operating with different spatial representations (e.g. networks, grids, polygons)
- Link models using different projections, units and categorizations
- Link models to other data sources (e.g. databases, user interfaces, instruments)
- Link new and existing (legacy) models with a minimum of reengineering
- Link models running on different platforms (e.g. Windows, Unix and Linux)
Framework for Risk Analysis in Multimedia Environmental Systems (Frames)
FRAMES is a software-based modeling system within which collections of models and modeling tools (e.g. data retrieval and analysis) are developed and applied to real world problems.
FRAMES allows models placed within its domain to communicate with each other, facilitating the passage of data, resulting in the simulation of complex environmental processes.
Additional Web Resources:
The capabilities of FRAMES are further discussed in another module:
Sensitivity and Uncertainty Analyses
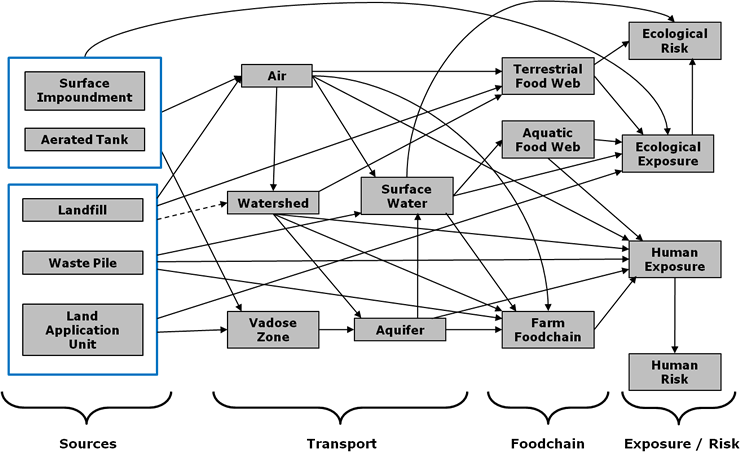
An example of the 17 science based models and their connectivity within FRAMES-3MRA. Adapted from EPA (2003). (Click on image for a larger version)
The 3MRA Example
Multimedia, Multi-pathway, Multi-receptor Risk Analysis (3MRA) is a set of 17 modules placed within FRAMES that collectively simulate the release, fate and transport, exposure, and risk (human and ecological) associated with waste-stream contaminants deposited in various land-based waste management units (e.g. landfills, waste piles).
Object Modeling System (OMS)
The OMS is an integrated environmental modeling system developed by Agricultural Research Service of the US Department of Agriculture (see Ahuja et al., 2005).
OMS is a computer framework consisting of:
- a library of science, control, and database modules;
- a means to assemble the selected modules into a modeling package customized to the problem, data constraints, and scale of application;
- an automatic generation of a friendly user interface;
- creation of a compiled, ready-to-run, version of the package.
The platform includes many interoperability features; such as the ability to handle all data types (file extensions) that can be translated for conversion or transformation as needed.
Additional Web Resource:
Advanced IT Infrastructure
The focus of this module has been on conceptual and computational approaches to integrated modeling. However, a large component of integrated modeling systems extends beyond linking models together; it also includes the "IT" side of integrated modeling.
Integrated modeling is more than the models and the science they represent. The logistics of integrating models is discussed further in the next section. However, the IT features of an integrated system might consider the bulleted items to the right.
Computational platforms or other integrated modeling IT infrastructures should be designed to handle:
- model connectivity;
- information architecture;
- framework connectivity;
- web-based access;
- system functionality;
- model evaluation and uncertainty analysis;
- education and knowledge management;
- achieving interoperability;
- automated data access, retrieval, and processing;
- the development of decision-support interfaces.
Challenges for Integrated Modeling
Notwithstanding the benefits of an integrated modeling approach from a scientific and decision making perspective, there are a number of challenges to advancing the state of the art and application of integrated modeling and environmental systems analysis (EPA, 2008).
These challenges can be categorized into three groups:
- Scientific or Knowledge-Based
- Information Technology (IT)
- Organization and Institutional
Fundamental topics are covered in other modules:
Scientific / Knowledge-Based Challenges
Potential science-related issues in the context of integrated modeling are: design of the integrated analytical framework,
model evaluation and uncertainty analysisuncertainty analysisInvestigates the effects of lack of knowledge or potential errors on the model (e.g., the "uncertainty" associated with parameter values) and when conducted in combination with sensitivity analysis (see definition) allows a model user to be more informed about the confidence that can be placed in model results., and education and knowledge management.
Linking multiple models together has its own set of challenges including: linking different model types (probabilistic vs. deterministic); reconciling different spatial and temporal scales; assessing uncertainty and finally applying the system of models in the correct context. Each component model, as well as the full system of integrated models, must also be evaluated (EPA, 2009b).
The practices for individual model development and evaluation discussed in other modules can be applied to integrated modeling. However, uncertainty analyses with integrated models become increasingly complex with an increased number of components (see Babendrier et al., 2005).
Information Technology Challenges
Achieving interoperability of many models, let alone two or three, is a significant challenge for integrated modeling efforts. The scientific understanding of the processes that link models from different media (e.g. air and water) forms the basis for integration; information technology (IT) provides the mechanistic means towards an integrated solution.
Some of the known issues facing integrating modeling from an IT perspective are: achieving interoperability; automated data access, retrieval, and processing; and the development of decision support interfaces (EPA, 2008).
There are at least three approaches to tackle the IT challenges of integrating models:
- Use of a software package framework designed for model integration such as FRAMES
- Following a set of model integration standards, specifications, and conventions such as OpenMI
- Hard-wiring of models without following any established standards
Organizational and Institutional Challenges
A significant hurdle for the promotion of integrated modeling into day-to-day practices has been stove-piping of research and modeling activities. Stove-piping of research, modeling and decision making activities is a systemic challenge that hinders effective and meaningful inter-office or inter-agency collaboration. Further, organizational structures do not often facilitate cross-media interactions.
Poor communication and collaboration also hinder cross-fertilization of ideas and true interdisciplinary research. However, integration of science and technology to support decision making is made possible when researchers, stake-holders, and decision makers from various sectors and disciplines come together and commit to share and implement ideas for a common purpose (EPA, 2008).
Collaboration is fundamental to integrated modeling. Groups of integrated modeling teams can collaborate to develop common solutions to common science and IT challenges. This in turn would facilitate further collaboration and reuse of integrated modeling systems. From continued collaboration, a community of modelers, stakeholders and decision makers could take form.
Community of Practice
In an effort to address these challenges and promote the benefits of integrated modeling, CREM has launched an international Community of Practice for Integrated Environmental Modeling (CIEM).
The CIEM aims to stimulate interactions among its members to foster learning and knowledge sharing and to spark innovation in the field of integrated environmental modeling. The CIEM will also promote integrated modeling as a means of achieving better management decisions, so that resources are used sustainably and broader impacts (often unknown during conventional, stove-piped approaches) are better understood.
One of the main goals of the CIEM is: "Leveraging the knowledge and tools of the community to advance integrated modeling science and technology."
The CIEM introduced the iemHUB to support their goals and the growing integrated environmental modeling community.
Additional Web Resource:
The iemHUB is an online community resource that supports the development, evaluation, and application of environmental models.
As a consequence of the interdisciplinary nature of environmental modeling, the iemHUB is designed to facilitate knowledge sharing, discussion and collaboration on models and tools that support multimedia and multidisciplinary analysis.
The iemHUB provides a unique environment for model access, simulation, and teaching and learning about environmental modeling.
Summary
Integrated modeling is not simply a model building exercise. From EPA (2008):
"...[integrated modeling] is a participatory methodology that can be used for gaining insight over an array of environmental problems spanning a wide variety of spatial and temporal scales."
The integrated modeling process includes multiple layers of integration and environmental systems analysis. EPA (2008) also identified essential qualities of integrated modeling.
Processes of Integrated Modeling:
- Development of conceptual models: Important components within each discipline should be identified and the relationships among these components (also across disciplines) should be characterized.
- Representation of information: Development of common data formats and underlying frameworks may facilitate interoperability.
- Support to decision makers: Understanding the specific needs of the decision makers may result in a more efficient model building process and may produce results that more directly support decision making.
- Stakeholder involvement: It is important that stakeholders be identified and engaged, incorporating their multiple sources of knowledge and information, identifying and mediating among their different priorities, and creating a shared vision.
You Have Reached the End of the Integrated Modeling 101 Training Module
From here you can:
- Continue exploring this module by navigating the tabs and sub tabs
- Return to the Training Module Homepage
- Continue on to another module:
- You can also access the Guidance Document on the Development, Evaluation and Application of Environmental Models, March, 2009.
References
- Ahuja, L.R., J. C. Ascough II, and O. David. 2005. Developing natural resource models using the object modeling system: feasibility and challenges. Advances in Geosciences 4: 29–36.
- Babendreier, J. E. and K. J. Castleton. 2005. Investigating uncertainty and sensitivity in integrated, multimedia environmental models: tools for FRAMES-3MRA. Environ. Model. Software 20(8): 1043-1055.
- Briggs, D. 2003. Making a Difference: Indicators to Improve Children’s Environmental Health. Geneva (PDF) (52 pp, 1 MB, About PDF) ExitWorld Health Organization.
- EPA (U.S. Environmental Protection Agency). 2000. Toward Integrated Environmental Decision-making (PDF)(64 pp, 529 K, About PDF). EPA-SAB-EC-00-011. Washington, DC. Science Advisory Board.
- EPA (US Environmental Protection Agency). 2003. Multimedia, Multipathway, and Multireceptor Risk Assessment (3MRA) System Volume I: Modeling System and Science. EPA530-D-03-001a. Athens, GA. Office of Research and Development.
- EPA (US Environmental Protection Agency). 2004. The Integrated Environmental Strategies Handbook (PDF)(192 pp, 10 MB, About PDF). EPA430-B-04-006. Washington, DC. Office of Air and Radiation.
- EPA (US Environmental Protection Agency). 2007. Hypoxia in the Northern Gulf of Mexico: An Update by the EPA Science Advisory Board. EPA-SAB-08-003. Washington, DC. Science Advisory Board.
- EPA (US Environmental Protection Agency). 2008. Integrated Modeling for Integrated Environmental Decision Making. EPA-100-R-08-010. Washington, DC. Office of the Science Advisor.
- EPA (US Environmental Protection Agency). 2009a. BASINS 4.0 Climate Assessment Tool (CAT): Supporting Documentation and User’s Manual. EPA/600/R-08/088F. Washington, DC. National Center for Environmental Assessment, Office of Research and Development.
- EPA (US Environmental Protection Agency). 2009b. Guidance on the Development, Evaluation, and Application of Environmental Models. EPA/100/K-09/003. Washington, DC. Office of the Science Advisor.
- Jakeman, A. J. and R. A. Letcher. 2003. Integrated assessment and modelling: features, principles and examples for catchment management. Environ. Model. Software 18(6): 491-501.
- Moore, R., Gijsbers, P., Fortune, D., Gergersen, J., Blind, M. (2007) OpenMI Document Series: Part A Scope for the OpenMI (version 1.4), HarmonIT. Exit
References (Continued)
- Ojeda-Martinez, C., F. Gimenez-Casalduero, J.T. Bayle-Sempere, C. Barbera-Cebrian, C. Valle, J. Luis Sanchez-Lizaso, A. Forcada, P. Sanchez-Jerez, P. Martin-Sosa, J. M. Falcon, F. Salas, M. Graziano, R. Chemello, B. Stobart, P. Cartagena, A. Perez-Ruzafa, F. Vandeperre, E. Rochel, S. Planes, and A. Brito. A conceptual framework for the integral management of marine protected areas. Ocean & Coastal Management. Vol. 52, Issue 2. Pages 89-101.
- Parker, P., R. Letcher, A. Jakeman, M. B. Beck, G. Harris, R. M. Argent, M. Hare, C. Pahl-Wostl, A. Voinov, M. Janssen, P. Sullivan, M. Scoccimarro, A. Friend, M. Sonnenshein, D. Barker, L. Matejicek, D. Odulaja, P. Deadman, K. Lim, G. Larocque, P. Tarikhi, C. Fletcher, A. Put, T. Maxwell, A. Charles, H. Breeze, N. Nakatani, S. Mudgal, W. Naito, O. Osidele, I. Eriksson, U. Kautsky, E. Kautsky, B. Naeslund, L. Kumblad, R. Park, S. Maltagliati, P. Girardin, A. Rizzoli, D. Mauriello, R. Hoch, D. Pelletier, J. Reilly, R. Olafsdottir and S. Bin. 2002. Progress in integrated assessment and modelling. Environ. Model. Software 17(3): 209-217.
- Parson, E. A. 1995. Integrated assessment and environmental policy making: In pursuit of usefulness. Energy Policy 23(4-5): 463-475.
- Risbey, J., M. Kandlikar, and A. Patwardhan., 1996. Assessing Integrated Assessments. Climatic Change 34: 369-395
- Rotmans, J. and M. B. A. van Asselt. 2001. Uncertainty Management in Integrated Assessment Modeling: Towards a Pluralistic Approach. Environ. Monit. Assess. 69(2): 101-130.
- Rosenberg, N. J. and J. A. Edmonds. 2005. Climate Change Impacts for the Conterminous USA: An Integrated Assessment: From Mink to the ‘Lower 48’. Climatic Change 69(1): 1-6.
- Smeets, E. and Weterings, R., 1999. Environmental Indicators: Typology and Overview. European Environment Agency, Copenhagen. Report No. 25, 19 pp.
- Tol, R. S. J. and P. Vellinga. 1998. The European Forum on Integrated Environmental Assessment. Environ. Model. Assess. 3(3): 181-191.
- Wenger, E. 1998. Communities of Practice: Learning, Meaning, and Identity. Cambridge University Press. 336 pages.
- Weyant, J. P., O. Davidson, H. Dowlatabadi, J. A. Edmonds, M. Grubb, E. A. Parson, R. Richels, J. Rotmans, P. R. Shukla, R. S. J. Tol, W. R. Cline and S. Fankhauser 1996. Integrated assessment of climate change: An overview and comparison of approaches and results. In. Climate Change 1995: Economic and Social Dimensions of Climate Change Contribution of Working Group III to the Second Assessment Report of the Intergovernmental Panel on Climate Change Eds. J. P. Bruce, H. Lee and E. F. Haites New York City, NY. Cambridge University Press. 368-396.
- Whelan, G. and G.F. Laniak. 1998. A Risk-Based Approach for a National Assessment. In: Risk-Based Corrective Action and Brownfields Restorations. C.H. Benson, J.N. Meegoda, R.B. Gilbert, and S.P. Clemence (eds.). Geotechnical Special Publication Number 82. American Society of Civil Engineers, Reston, Virginia, pp. 55-74.